How do coastal wetlands respond to rising sea levels? Will they survive the ocean’s accelerating rise along our coastlines? How can we know? What can we do to conserve these important ecosystems?
High school curricula from Stroud Water Research Center and partners allow educators to explore these questions and more with their students.
Coastal wetlands are complicated and dynamic systems, and science has not yet been able to predict their future. They offer critical benefits to land-based, coastal, and marine systems. From providing organic matter to sustaining food webs to providing habitat for birds and other wildlife, the ecosystem services of coastal wetlands are significant. Yet they struggle to attain recognition that can fuel efforts to protect them from sea level rise and human development.
These benefits and challenges make coastal systems a rich tool of real-world scientific investigation upon which to build curriculum.
Rivers carry sediment to and through coastal marshes, but the quantity of sediment, variation over time, and sources of that sediment are not well understood. Yet this sediment may partly determine the fate of the marsh as oceans continue to rise. Stroud Center scientists have identified ways to trace the origins of sediment in coastal rivers, a key tool for determining how the sources contribute to marsh stability. They have also been able to harness the molecular characteristics of the microbes attached to the sediment particles to trace the sediment source.
With a group of experienced teachers and curriculum developers, Stroud Center educators have developed high school curricula to engage your students in this fascinating and complicated real-world problem!
Why Do We Care About Wetlands?
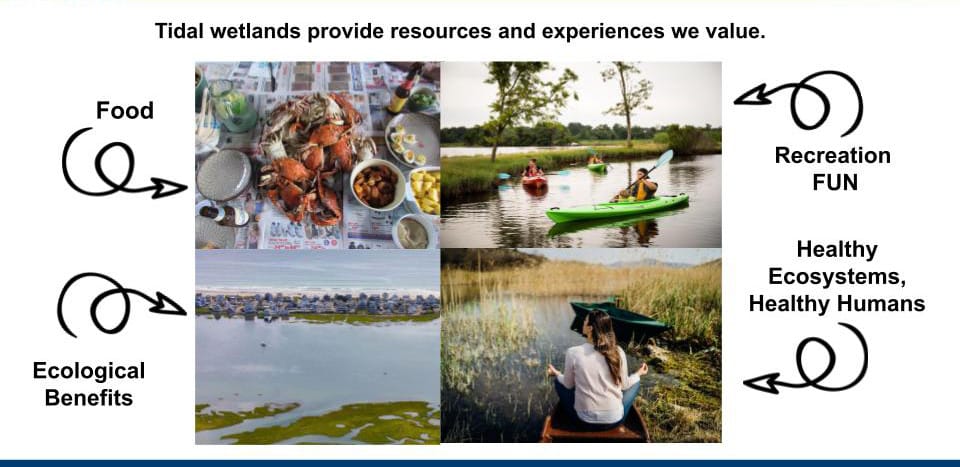
Tidal wetlands, from salt marshes to cypress swamps, are not just a scenic backdrop for coastal lifestyles. These important ecosystems are home to diverse animal and plant communities, provide valuable outdoor experiences for people, and link biogeochemical cycles occurring in watersheds and their estuaries. Tidal wetlands are also shifting and changing as rising sea level pushes inland, in some places disappearing under the tide forever or migrating upslope with altered plant communities. While the recent acceleration of sea level rise is driven by global warming, the response of tidal wetlands is governed by plant physiology, hydrodynamics, and sediment supply.
Measuring the sand, silt, and mud carried by rivers and delivered to tidal wetlands is more complicated than it might seem. Scientists are using high-tech methods borrowed from the biotechnology sector to track where sediment comes from. Measurements over short timescales (like individual tidal cycles) to long timescales (like decades) help predict how tidal wetlands in different places will respond to sea level rise. But not everyone needs to use these tools to appreciate the balancing act playing out in tidal wetlands across the coast: a few simple lessons can help people understand these processes and their implications for our watersheds, our communities, and how we can live sustainably on our coasts.
When Sea-Level Rise Threatens Coastal Wetlands, Don’t Look to Rivers For Help, Scientists Say
Building up wetlands that are drowning under rising oceans remains a challenge, but scientists are now one step closer to identifying solutions. Amid climate change, large dam removal projects have gained attention as a solution to the loss of coastal wetlands that reduce flooding, filter water, and provide wildlife habitat. But in a paper in Science, researchers concluded this strategy won’t work in most U.S. rivers.
Meet the Scientists
Learn More About the Project
- Read about the wetlands study.
- Read a research paper on this project and discuss it with your students!
- Watch a video with an introduction to the project as well as Dr. Ensign’s career journey.
High School Curricula Created by Teachers in Pennsylvania and Maryland
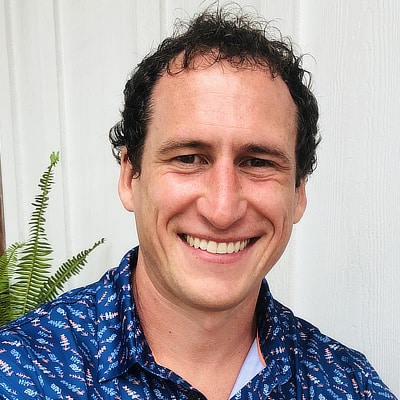
Anthony Prinzo (Pennsylvania, USA): I am a biology teacher and the science department chair at East High School in the West Chester Area School District. With a bachelor’s degree in marine biology and a master’s degree in secondary education in biology, I have taught all levels of biology as well as human anatomy, aquatic ecology, marine biology, and environmental science over the past 10 years. I am most passionate about the aquatic ecology and marine biology classes because it is an opportunity to teach about the estuaries, the environment, and other concepts students may have experience with. This is what I recommend for all teachers jumping into estuary education. Make it relevant for our students. Tap into their life experiences, and they will be interested in the topic.
Prinzo produced the “Sea Level Rise and Tidal Wetlands” curriculum in partnership with Stroud Water Research Center, supported by National Science Foundation Award No. 2049073.
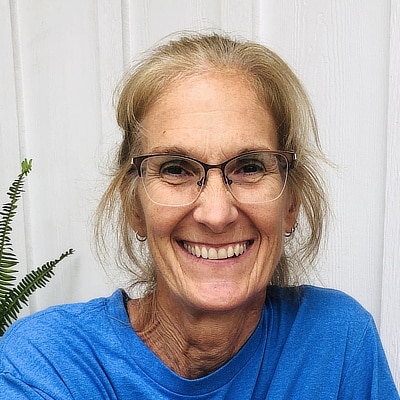
Marie Schneider (Pennsylvania, USA): After 17 years in the environmental field, I changed careers and became a high school biology teacher. Best move ever! Witnessing students explore and engage in science is so rewarding. In addition to biology, I teach environmental science, aquatic ecology, marine biology, oceanography, and meteorology. I earned my bachelor’s degree and master’s degree from West Chester University, both in biology. I am a self-proclaimed tree hugger, and I’m happiest when I’m experiencing nature. Exposing students to the natural world and having them recognize the importance of complex interactions is a role I do not take lightly. I find this especially true in aquatic ecosystems like wetlands and estuaries. It’s easy to look at these areas and dismiss them as unimportant, but once students begin to explore what is beneath the surface (both literally and figuratively!), they learn to appreciate the many benefits we often take for granted. This leads to environmental stewardship, and what could be more rewarding than that?
Schneider produced the “Tidal Wetlands and Climate Change” curriculum in partnership with Stroud Water Research Center, supported by National Science Foundation Award No. 2049073.
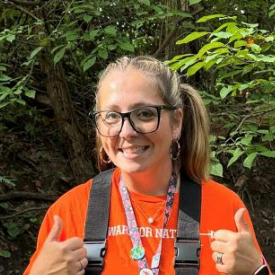
Lauren Rose (Maryland, USA): I graduated from Shippensburg University in 2008 with a bachelor’s degree in biology and then went on to earn a master’s degree in chemical and life sciences from the University of Maryland. After graduation, I began working as a science teacher at Easton High School, where I taught a variety of science classes for 16 years, serving as department chair for five of those years. In 2023, I received the Environmental Protection Agency Region 3 Presidential Innovation Award for Environmental Educators. In 2024, I received the Outstanding Young Alumni Award from Shippensburg University.
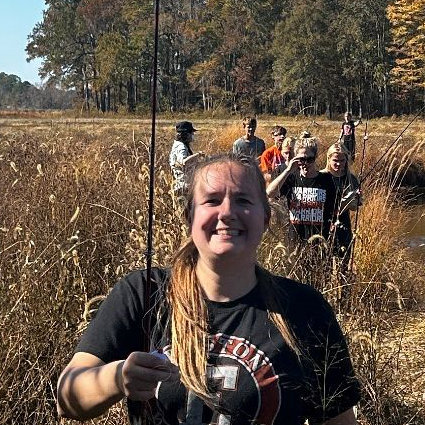
Kelly VanTrieste (Maryland, USA): I am a science teacher at Easton High School in Easton, Maryland. I currently teach freshmen biology, AP biology, and a sheltered Earth Systems class for newcomer students. I went to West Chester University, where I earned my degree in Biology Education (Go RAMS!). I earned my master’s in Teaching English to Speakers of Other Languages (TESOL) at Salisbury University. Growing up in southeastern Pennsylvania, I was not exposed to the Chesapeake Bay region and its wonders. Since moving to Maryland, I have developed a deep appreciation for the Chesapeake Bay region and all it has to offer. Experiencing the wonders of the region has opened my eyes to the importance of environmental education and getting students outside to foster their love for nature.
Rose and VanTrieste produced the “Modeling How Scientists Study Wetlands in Climate Change” curriculum in partnership with ShoreRivers and Stroud Water Research Center, supported by National Science Foundation Award No. 2049073.
Terms and Definitions
- Accretion: The vertical accumulation of material on the surface of a wetland.
- Bulk density: The mass per volume of wetland sediment, such as kilograms per cubic meter.
- Estuarine turbidity maximum: A zone of perpetually-elevated suspended sediment in an estuary.
- Estuary: A semi-enclosed body of water with tidal influence.
- High-throughput sequencing: An automated way to rapidly determine the genetic sequence of all DNA or RNA present in a sample.
- Mixing model: A calculation of the proportion of material in a sample derived from known sources.
- River sediment load: Mass of sediment per unit time, such as cubic meters per second.
- Sea level rise: Vertical rise of average sea level due to land subsidence, thermal expansion of water, ice melt, and slowing ocean currents.
- Shear stress: The hydraulic force exerted on a particle resting underwater; when a critical threshold is exceeded, the particle will move.
- Source tracking: An exercise to determine where material in rivers, like sediment, comes from over a certain time period.
- Suspended sediment: The mineral and organic detritus maintained in the water column of a river by shear stress and turbulence.
- Tidal river: The zone of an estuary that may not have salt and resembles the meandering character of streams with adjoining forests.
- Tidal wetland: A terrestrial habitat dominated by hydric soils, hydrophilic vegetation of any kind, and regular inundation by tides.
- Turbulent flow: The opposite of laminar flow in which eddies of varying sizes continuously mix a fluid and dissipate energy.
- Watershed sediment yield: The mass per area per time (e.g., kilograms per square kilometer per year) exported from a land surface.
This material is based upon work supported by the National Science Foundation Division of Earth Sciences under Grant No. 2049073. Any opinions, findings, conclusions, or recommendations expressed in this material are those of the author(s) and do not necessarily reflect the views of the National Science Foundation.