This project was based on research conducted by scientists at Stroud Water Research Center that shows the connections among landscape practices, stormwater runoff, and the mobilization and mineralization of carbon. It also built upon sound landscape practices used at Longwood Gardens to teach visitors that they can get beautiful results when replacing lawns and paved surfaces with land cover that allows for rainwater infiltration.
What is an Ecosystem Esthetic?
In the final chapter of A Sand County Almanac, Aldo Leopold discussed the relationship of humans to their environment and proposed the adoption of a “conservation esthetic,” that idealizes recreation in which humans are part of the natural world. We have expanded this idea to include our vision of an “ecosystem esthetic” in which human activities on the landscape facilitate ecosystem processes.
What is an ecosystem esthetic? Let’s start by breaking down the term and understanding each piece.
- Ecosystem: an assemblage of plants, animals, and microogranisms and their physical and chemical environment functioning as an ecological unit.
- Esthetic: a particular theory or conception of beauty.
Ecosystem-level processes include the flow of energy (think of how the energy from the sun is first captured by plants which are then eaten by animals or decomposed by microorganisms) and cycling of nutrients (as when an atom of nitrogen or phosphorus in a decaying tree stump is returned to the soil and used to nourish a new seedling). With an ecosystem esthetic, we value landscapes that preserve natural processes, we perceive their natural beauty, and recognize the beauty of the processes themselves. When we include an understanding of energy flows, water cycling, and nutrient cycling as fundamental components in our land use decisions, we can make better decisions for ourselves and the planet.
Changing Notions of an Ideal Landscape
Man’s Dominion Over Nature
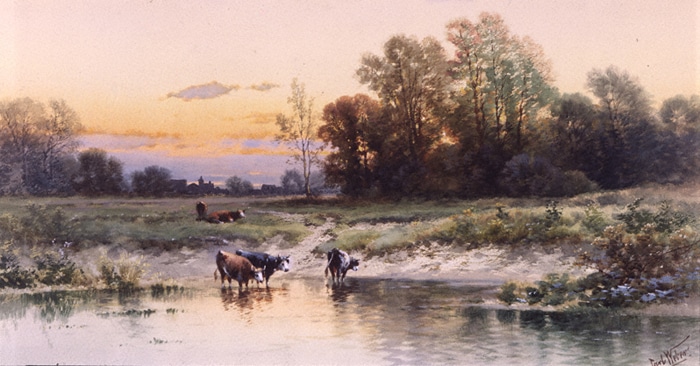
The concept of man’s dominion over nature, in the Western world, is found in the book of Genesis, but whether dominion refers to stewardship or subjugation is a matter of debate. The 17th Century Puritans believed that Nature was God’s gift to man. In 18th Century Colonial America, Thomas Paine popularized the Deist ideal that God is revealed in nature in his pamphlet, The Age of Reason. By the 19th Century, prominent literary figures including Emerson and Thoreau espoused Transcendentalism and a belief in the inherent goodness of man and nature.
These intellectual and religious currents likely influenced popular romantic views of nature captured by painters like Carl Weber. Here the hand of man, manifest in forested lands cleared for agriculture and streams as watering troughs for livestock, figure prominently into the ideal landscape.
Similarly, in his painting of Gilpin’s Mill on the Brandywine, Thomas Doughty celebrates harnessing the power of Nature to serve industrial development in Colonial America.
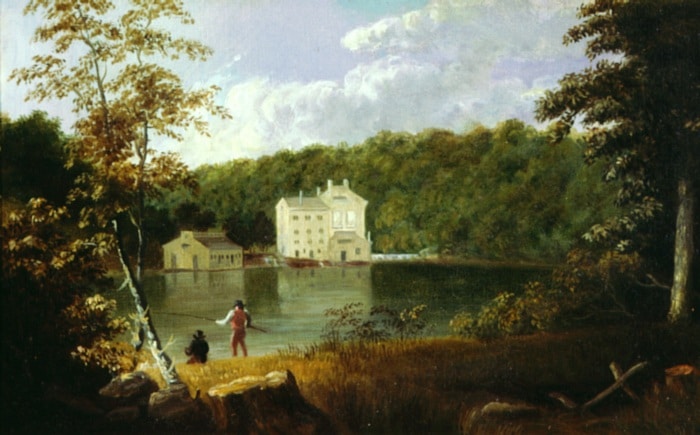
Suburban Lawns Proliferate Following World War II
“A fine velvety carpet of grass is a work of art that is one of the finest products of nature. The growing of a luxurious weedless turf is profitable from every view point…” — Albert A. Hansen, Purdue University Agricultural Station, 1928
The front-lawn esthetic in the United States, at first an emulation of English estates and then influenced and spread by the designs of Frederick Law Olmsted, was part of American culture in the mid-19th Century. The trend, supported by garden clubs and research by the U.S. Department of Agriculture on turf grass propagation popularized American lawns, but was interrupted by the Depression and WWII.
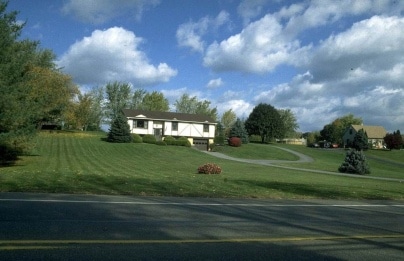
Following the war, the growth of suburban developments led to a boom in lawns that was described in the Saturday Evening Post as “… an emotion that has blossomed into a status symbol.” A multi-billion dollar lawn care industry exists in the United States today and the turf grass lawn reigns supreme as the ideal American landscape for homeowners.
For detailed discussions of the American lawn, see the following books:
- The Lawn: A History of an American Obsession. 1994. Virginia Scott Jenkins. Smithsonian Institution Press.
- Redesigning the American Lawn: A Search for Environmental Harmony. 1993. F. Herbert Bormann, Diana Balmori, and Gordon T. Geballe. Yale University Press.
- Second Nature: A Gardener’s Education. 1991. Michael Pollan. Grove Press.
The New “Lawn” As Livable Landscape
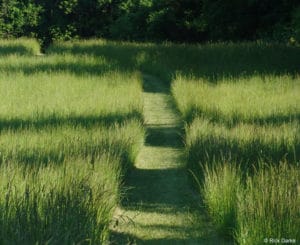
A change from turf grass dominated landscapes to more naturalized landscapes has been informed by concern over the use of lawn chemicals, knowledge that diversity can impact the sustainability of plantings, and a desire to create a habitat more conducive to wildlife. It is unclear whether some of the desire to move away from turf grass is based upon a changing esthetic that includes environmental concerns, an evolving ideal of what is beautiful, or some combination of the two.
While this may seem like a new idea, 32 years ago Rene Dubois wrote in Celebrations of Life “For many people, their lawns provide a clear opportunity to think globally, but act locally.”
The Science Behind the Esthetic
The Carbon Cycle and Climate Change
When scientists consider how humans impact the planet, they often focus on biodiversity (the variety and distribution of life forms inhabiting an area) and eutrophication (over-fertilization or the enrichment of an ecosystem with excess nutrients).
These are important topics. However, we feel it is necessary to reframe the discussion and put global carbon cycles at the center. Why? Because climate change is real, caused by humans, and is related to the global carbon cycle. (View Berkeley Earth’s analysis of land-surface temperature records going back 250 years).
The Basic Carbon Cycle
Inorganic carbon in the form of carbon dioxide is removed from the atmosphere by plant photosynthesis and converted into organic carbon in the form of plant biomass. Carbon is returned to the atmosphere through respiration, including the respiration of microorganisms involved in the decay of non-living plant materials.
Human burning of non-living organic carbon in the form of fossil fuels, has added carbon dioxide to the atmosphere. Carbon dioxide and other greenhouse gases trap heat radiating from the Earth back into space, warming the Earth.
Let’s take a look at how scientists have used past climate data to develop models that accurately capture the dynamics and magnitudes of global temperature changes and then apply those models to predict the future. In the temperature anomalies chart, notice how well the model (gray) matches the recorded data (red). The ability to replicate historical temperatures or warming demonstrate the accuracy of the model.
Predictions For the Next Century
Predicting the future, however, becomes more difficult, because so much of the future climate state depends upon human activities; human actions are the source of uncertainty. Models tell us that, with no change in human activities over the next 100 years, carbon dioxide will increase by 200-300% and will trigger global warming of around 3°C (5.4°F).
In the history of the Earth, a temperature change of that magnitude has never happened in less than 5,000 years. In an alternative scenario, if carbon dioxide increases can be held to 70%, global warming would be less than 1°C.



Carbon Movement Through the Landscape
Most people are familiar with how carbon dioxide in the atmosphere traps heat and is related to climate change. Let’s look at how carbon travels through plants and soils to the atmosphere, rivers and seas.
Through Plants and Soils
Plants use sunlight and water to remove carbon dioxide from the atmosphere through photosynthesis. This is the ultimate source of most of the organic carbon in terrestrial ecosystems. Some of the fixed carbon is returned to the atmosphere as plants respire and some is returned to the atmosphere by microbial respiration of fallen leaves and other plant materials that end up on or in the soil.

From Plants and Soils Through Streams and Rivers
Some organic carbon from the landscape enters streams when leaves or other plant materials fall in or are blown in, but most of the terrestrial organic carbon gets to streams as molecules dissolved in rain water or ground water. This dissolved organic carbon from the landscape provides most of the useful energy for stream life and in most streams exceeds the organic carbon entering streams as leaf litter or entering stream water when algae on the stream bed carry out photosynthesis.
Into the Oceans and Back to the Atmosphere
Organic carbon, in the form of dissolved molecules or as solid particles is carried downstream by the currents. Some of the carbon is respired by microorganisms in the stream and some is buried. Along the path to the oceans, some respired carbon moves back into the atmosphere through a process called CO2 evasion.
In this way, carbon moves through the landscape, connecting the atmosphere, the biosphere, and the aquatic environments.
So where do we find the greatest concentrations of carbon? Outside of the Earth’s uppermost mantle and crust (rocks or the lithosphere), most of the carbon is in the ocean and in soils. Setting aside the carbon in the lithosphere, it breaks down like this:
- 1% in land plants,
- 2% in the atmosphere,
- 6% in soils, and
- 91% in the oceans
Astonishingly, the lithosphere contains about 1000 times more carbon than the oceans, including carbon in coal, gas, oil, and limestone.
The Role of Soils in the Carbon Cycle
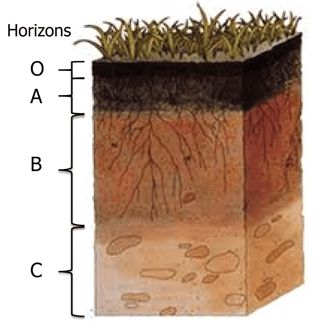
When you stand in your landscape, most of the carbon is not in the plants around you or the air above you, but under your feet.
In fact, soils contain nearly three times as much carbon as the atmosphere, and more than four times as much as all land plants combined. Some of the organic carbon in the soils is rapidly respired by soil microorganisms in just a few days, but some soil carbon, especially the carbon associated with mineral surfaces, lasts for years, decades, centuries, and even millennia.
The Deeper You Dig, the Rockier It Gets
Although soils contain a great amount of organic carbon, most of it is near the surface. The amount of organic carbon declines with soil depth.
As any gardener knows, there is a thin layer of organic material (O) at the top of a soil horizon. Below that is a layer of minerals mixed with organic remains (A). Organic carbon continues to decline through a layer of minerals mixed with iron and aluminum oxides and clays (B) and finally to a layer of unconsolidated materials (C).
Natural soil processes are very important in the carbon cycle, and we’ll return to that point in a moment.
How is Carbon Moved By Water?
We’re all familiar with the basic hydrologic cycle, right? Water in the atmosphere forms clouds, precipitation falls to the ground where portions run off into water bodies or seep into the soil and are taken up by plants which move some of the water back into the atmosphere through evaporation and transpiration to start the cycle over, and some of the soil water continues moving downward into the ground water that supplies stream flow during periods without rain.
Through the Trees and Into the Soil
Carbon goes along for the ride. Precipitation picks up organic carbon as it moves through the tree canopy and through the forest litter layer. Some of the carbon is washed off of surfaces, while some is extracted from plant matter, much like carbon is extracted from a tea bag as it steeps.
After picking up organic carbon on its way to the soil, water loses carbon as it moves down through the soil. Organic carbon dissolved in the water moves through pore spaces between soil particles or through macropores, channels through the soil caused by earthworms and other animals, or when tree roots die and decay. Some of the dissolved organic carbon combines with metal oxides in the soil and sticks to clay particles. This process allows natural soils to sequester organic carbon and reduces the amounts of organic carbon in ground water.
Water Flowing Overland and Underground
Some of the particles of organic carbon in soils and the dissolved organic carbon associated with the surfaces of soil particles is consumed by microbes and then released as carbon dioxide when the microbes respire. The respired CO2 can be trapped in the soil preventing it from returning to the atmosphere even when concentrations in the groundwater become supersaturated. When some of the ground water eventually moves back to the surface in streams it typically has low concentrations of organic carbon and high concentrations of CO2. As ground waters enter streams, some of the CO2 outgasses into the atmosphere.
Some precipitation takes a different path, flowing overland through the organic carbon rich litter layer, and picking up even more organic carbon before it enters streams and rivers. Clearly, the path the water takes in getting to a stream influences the amount and types of carbon it contains.
The Importance of “River Breath”
Scientists used to think that streams were like pipes, moving carbon from land to ocean. In fact, the Intergovernmental Panel on Climate Change did not consider freshwaters in their analysis of the global carbon cycle. In 2009, Stroud Water Research Center scientists collaborated on a paper, The Boundless Carbon Cycle, that argued that inland waters are very important to the global carbon cycle, with losses and possibly sequestration through burial in floodplains and reservoirs and inputs through outgassing.
Research is showing that “river breath” from tropical rivers outgasses more carbon to the atmosphere than they discharge to the oceans — ten to fifteen times more carbon! And these estimates are actually being revised upwards. Most outgassing of carbon occurs as CO2, but some carbon from lakes, wetlands, and especially reservoirs can be released as methane, a potent greenhouse gas that traps heat more efficiently than CO2.
How Do Humans Impact the Carbon Cycle?
What happens when we cut down forests and replace them with structures, pavement, and lawns? The natural hydrologic cycle is severely impacted.
Natural Cycle: Rainfall on a Forest
Most of the rain that falls on a forested landscape moves into the soil. From there it either moves back into the atmosphere or into groundwater.
This process allows the soils to sequester (remove) carbon from the water. It also replenishes the aquifers that many of us rely on for drinking water and that streams rely upon for flow when it is not raining.
Impacted Cycle: Rainfall on a Developed Landscape
When rain falls on an urbanized landscape, nearly all of the rainfall runs off! Why?
- Paving materials that are not special porous paving do not allow water to move into the ground.
- Urbanization impacts the physical structure of soils; regraded and compacted soils keep water from percolating down.
- Fewer plants mean that very little water is moved back into the atmosphere.


Asphalt and Concrete Aren’t the Only Problems
It’s easy to understand why rainfall moves through undisturbed soils easier than through concrete: woodland soils are much less dense than concrete.
But what about all of the “green space” in our communities?
Unfortunately, soils in residential neighborhoods, golf courses, parks, and athletic fields are typically compacted. This increases the bulk density of soils which allows less water into the soil than do forested lands and also results in less soil carbon.
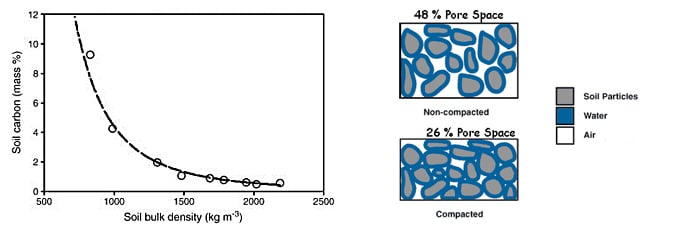
The impact on hydrology: lawns “shed” lots of water.
Less water makes it into the ground, which means less water to replenish the aquifers.
It also means that instead of allowing carbon to be sequestered in the soil, the carbon is now swept into streams with all of the runoff.
WikiWatershed
Stroud Water Research Center has developed the Model My Watershed web apps to demonstrate how land use and soil structure influence runoff. The web apps are part of the WikiWatershed toolkit.
- Model My Watershed lets you analyze real geo-data, model storms, and compare conservation or development scenarios in a watershed.
- Model Micro Site Runoff lets you explore how land use and soil determine runoff for the Site Storm Model package of Model My Watershed.
What’s So Bad About Carbon in Streams?
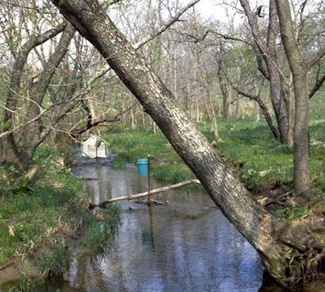
It is a question of balance. Most of the biologically useful energy for life in streams comes from the land and most of it is present as dissolved molecules. These dissolved molecules fuel stream life including microorganisms that consume the oxygen in the water.
So, increasing the carbon inputs to inland waters means decreasing the oxygen dissolved in water that many life forms reply upon, increasing “river breath”, or outgassing, to the atmosphere, and making the water more difficult to treat with disinfectants for safe drinking water.
Water Flux Versus Carbon Export Flux
Looking at these dramatic photos of a stream during baseflow and stormflow, you might think that most of the water flow in a stream happens during a storm.
Actually, a stream moves the bulk of its water during non-storm conditions and small rainfall events. 92% of rainfall happens during storms with 2 inches of precipitation or less over 24 hours.
However, while water flux is dominated by baseflow, the majority of carbon exports in a stream happen during storms. That’s one reason stormwater management is so important.
Stormwater Management and Carbon Exports
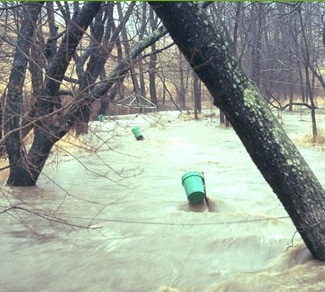
As we learn more about how storms move carbon, the goals of stormwater management have changed to incorporate:
- Flood control
- Water quality
- Groundwater recharge
Better soil conservation practices and better stormwater management keep more carbon out of streams and reduce losses to the atmosphere.This is because better stormwater management means increasing infiltration — allowing natural soil processes to sequester organic carbon in stormwater.
Increased infiltration, in turn, means less runoff, which reduces the carbon flux downstream during storms and therefore reduces losses to the atmosphere and accumulations in estuaries and coastal waters.
Reducing Your Landscape’s Carbon Footprint
The American lawn of turf grass has uses that are difficult to replace with other landscapes, and an ecosystem esthetic is not anti-lawn, but rather a recommendation to reduce the size of your lawn.
Data that compare C sequestration in soils supporting turf grass versus landscapes that contain a mixture of turf grass, meadow grasses, and woody vegetation are difficult to obtain. It is even more difficult to obtain information on the ages of soil carbon under these different land use scenarios. Using an estimate that turf grass soils store 7 kg C/m2 and forest soils store 12 kg C/m2, this difference of 5 kg C/m2 on a 1 acre plot equates to the carbon foot print of driving a car about 50,000 miles based on the U.S. EPA Greenhouse Gas Equivalencies Calculator.
We’ve told you why it’s important to reduce the carbon exports from your landscape. What are the simple actions you can take?
- Reduce the size of your lawn
- Encourage a diversity of plants in your yard and start with native plants. An ecosystem esthetic does not exclude the use of exotic plants, but strongly encourages the use of native plants. For detailed discussions about the benefit of native plants see the recent work of Douglas Tallamy, Bringing Nature Home: How You Can Sustain Wildlife with Native Plants, 2007, Timber Press. For a historical perspective on the use of native plants and a naturalistic approach to gardening, see the recent expanded edition of the 1895 fifth edition of The Wild Garden by William Robinson, with new material and images by Rick Darke that place Robinson’s work in a modern context: The Wild Garden: Expanded Edition by William Robinson and Rick Darke, 2009 Timber Press, Portland Oregon.
- Minimize impervious surfaces
- Compact natural soils as little as possible and consider using deep ripping techniques to reduce the bulk density.
To learn more about soil compaction and its effects, download:
- Urban Soils, Part 1: Understanding Compaction
- Urban Soils, Part 2: Measuring Compaction
- Comparing Silva Cells and Structural Soil
- Investment vs. Returns for Healthy Urban Trees: Lifecycle Cost Analysis
Photo Gallery
You’ve learned about the science behind the ecosystem esthetic and why your landscape choices are so important. The good news is that your landscape can be a thing of beauty and make a difference, as demonstrated by these images of beautiful, livable landscapes.
All photos are by Rick Darke. Click on a photo to enlarge.
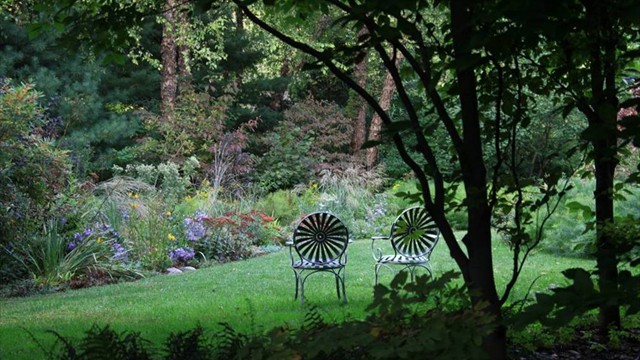
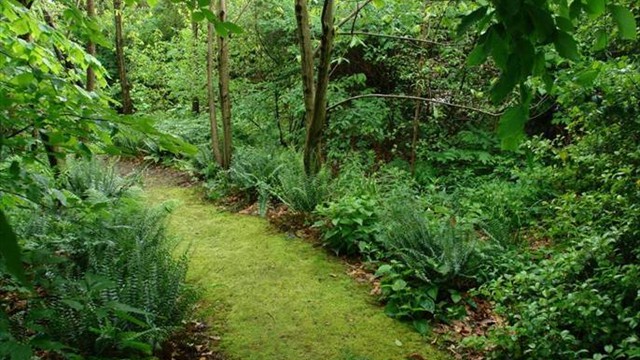

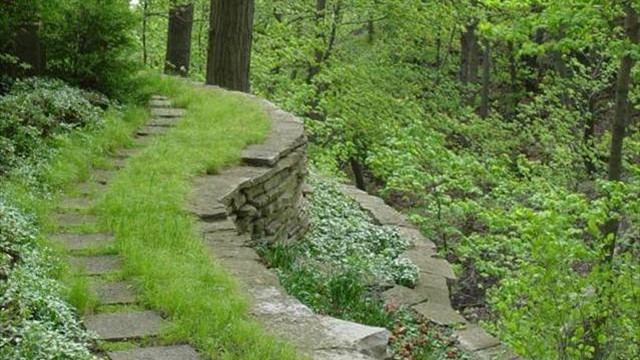

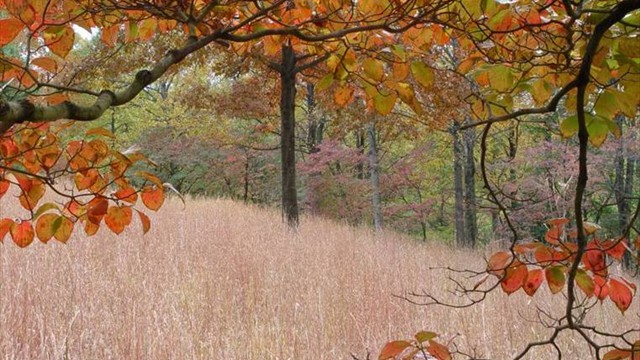




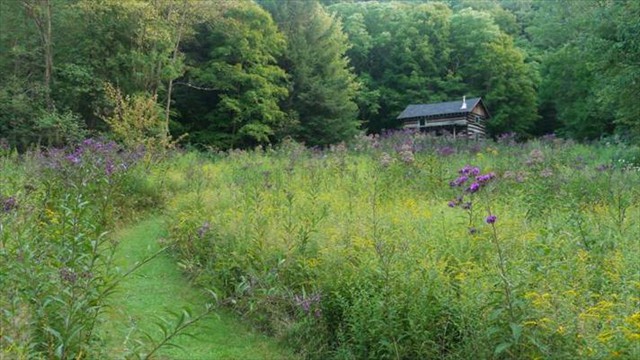




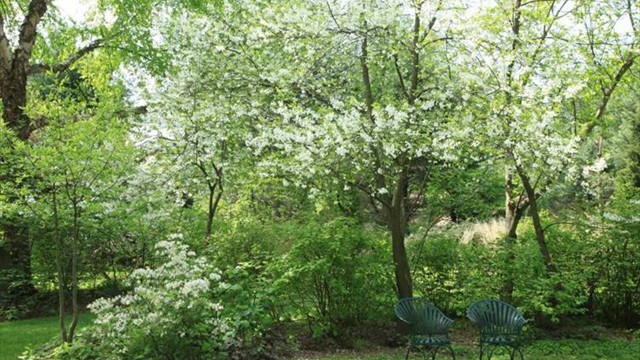

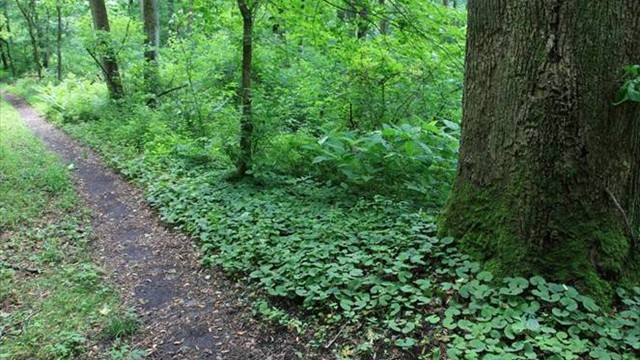
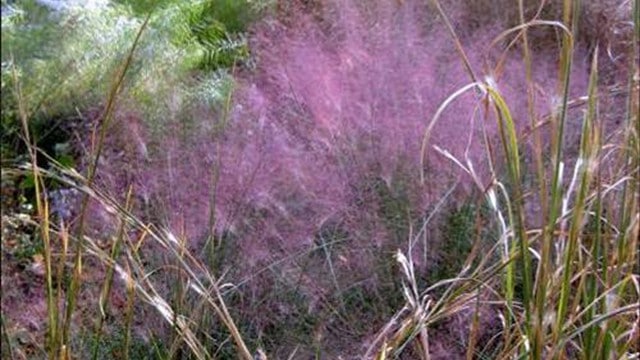




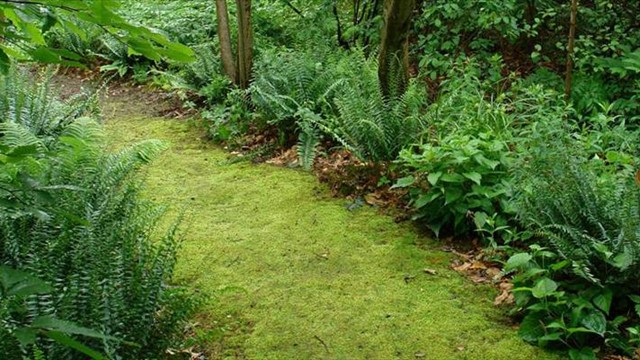
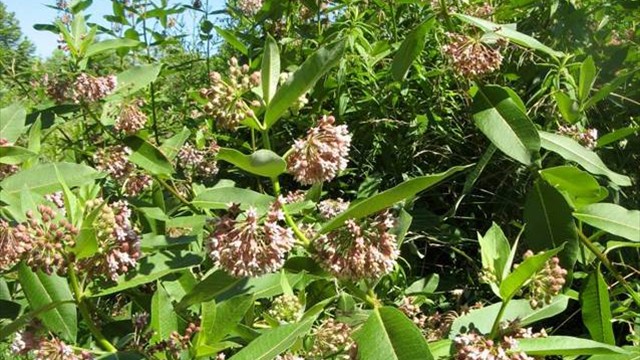
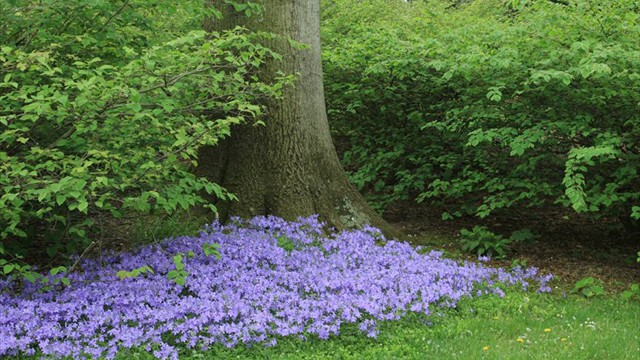

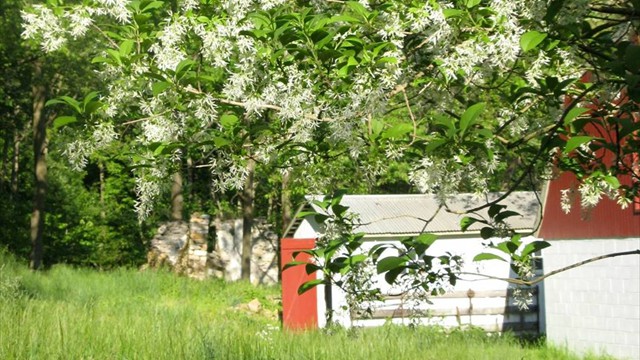

Contributors
Michele Adams, P.E.
Ms. Adams is the president and founder of Meliora Design. For more than 28 years, her work has encompassed environmentally sensitive site design and sustainable water resources engineering. Building on a multi-disciplinary approach, her work includes both master planning and design for campuses, cities, urban restoration projects, commercial, industrial and residential installations, public facilities, and environmental education centers. In all her work, Ms. Adams seeks to combine sound engineering science with an understanding of natural systems. She is a frequent lecturer and educator on the topics of water and sustainability, and has provided technical expertise to clients ranging from watershed advocacy organizations to corporations. Ms. Adams was one of the principle authors of the Pennsylvania Stormwater Manual, and serves on the U.S. Green Building Council’s Technical Advisory Group for Sustainable Sites, and American Rivers Science and Technical Advisory Committee. She frequently serves as an expert witness with regards to stormwater and surface water quality issues.
José Alminaña, FASLA, LEED® AP
José joined Andropogon Associates in 1983 and has been a principal since 1995. Trained as a landscape architect and architect, José excels at thinking outside the box, while still unearthing the realities of innovative construction techniques. With a passion for detail, José strives to create sensitive, sustainable designs that respond directly to each site’s unique environmental conditions. He has directed a diversity of design and planning projects, from restoring urban parks and designing corporate campuses to planning new mixed-use communities. Regardless of project scale, José distills the site’s essential resources into designs that are functional, beautiful, and environmentally responsible. José is a visiting lecturer at Drexel University and the University of Pennsylvania School of Design.
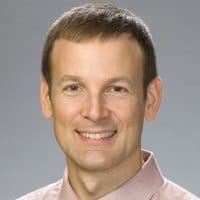
Anthony K. Aufdenkampe, Ph.D.
Stroud Water Research Center
Research Interests: investigating the processes of organic matter cycling throughout watersheds — from soils to rivers to estuaries; elucidating the interplay between degradation and sorption processes in determining the composition and ultimate fate of organic matter within river systems; employing diverse analytical and experimental techniques to answer hypothesis-driven questions; synthesizing observation and theory into quantitative models of various types in order to guide new experiments and theory.
Rick Darke
Rick Darke is a widely published author, photographer, lecturer and consultant focused on regional landscape design, planning, conservation, and enhancement. Blending art, ecology, and cultural geography, Darke is dedicated to the design and stewardship of the livable landscape. He has studied and photographed North American plants in their habitats for over 30 years, and this work is reflected in his articles and books including The American Woodland Garden: Capturing the Spirit of the Deciduous Forest.
A broadly knowledgeable horticulturist, Darke has traveled extensively in both hemispheres, exploring diverse ecologies and cultural landscapes in search of ideas to enrich the global garden. He is an internationally recognized authority on the use of grasses in designed and managed landscapes and his book, The Encyclopedia of Grasses for Livable Landscapes is the world’s most complete individual reference on this topic. His book The Wild Garden: Expanded Edition, places William Robinson’s classic concept of wild gardening in modern ecological context and illustrates its relevance for today’s gardeners and landscape stewards. Darke’s writing and photography is included in The New American Landscape: Leading Voices on the Future of Sustainable Gardening and Fallingwater. Darke’s writing and photography is featured in On The High Line: Exploring America’s Most Original Urban Park. Visit Books By Rick Darke for more information.
Susan E. Gill, Ph.D.
Stroud Water Research Center
Gill came to education through a circuitous route. As an undergraduate, she studied environmental planning. She earned an MA in environmental education at Beaver College and then a Ph.D. in geology at the University of Pennsylvania (UPENN). Gill directed the Master of Environmental Studies at UPENN and taught several courses for the program. She moved to Stroud Water Research Center in 2007 as Director of Education. She worked on developing watershed tools through the WikiWatershed.org portal. The first of these applications is Model My Watershed®, an online, geospatial hydrologic model that uses real data to calculate hydrology in neighborhoods in southeastern Pennsylvania and northern Delaware. Gill retired in 2015.
Louis A. Kaplan, Ph.D.
Stroud Water Research Center
Research Interests: Organic matter biogeochemistry, aquatic microbial ecology and nutrient cycling, especially the characterization and quantification of biodegradable dissolved organic carbon and the structure of microbial communities in stream ecosystems.
Muscoe Martin, AIA, LEED®
Muscoe Martin’s 25-year career reflected his passion for and commitment to ecological design. Prior to founding M2 Architecture, Muscoe was a Senior Associate at Wallace Roberts & Todd, and from 1996-2002, he was a Principal at Susan Maxman & Partners where he managed a number of award-winning “green” projects. Muscoe was a nationally recognized expert on green design, an appointed member of the USGBC’s LEED® Steering Committee, and chairman of the LEED® Market Advisory Committee. He was also a member of LEED® Faculty and a Lecturer at the University of Pennsylvania School of Design where he taught a course on Ecological Design. Martin passed away in 2014.